The dual role of mesenchymal stem cell-derived exosomes modulates the phagocytic activity of macrophages and promotes cardiac recovery after heart attack
The World Health Organization considers ischemic heart disease (IHD) as the leading cause of death worldwide, accounting for 16% of total deaths worldwide (https://www.who.int/news-room/fact-sheets/detail/the-top-10-causesof-death). Since 2000, the largest increase in deaths has been for this disease, rising by more than 2 to 8.9 million deaths in 2019 (1). The most prevalent clinical manifestations and complications of IHD are acute myocardial infarction (AMI), cardiac arrhythmias, recurrent myocardial infarction (MI), heart failure (HF) and cardiac arrest (2). Current knowledge about the risk of recurrent MI and approved therapies do not acceptably reduce the threat to patients surviving a second AMI (3). Cardiac remodelling following an ischemic event leads to adverse phenomena resulting in progressive HF (4). In particular, the inflammatory response triggered by the release of danger factors from necrotic cardiomyocytes drives the migration of inflammatory cells into the compromised tissue (4,5). The resolution of the inflammatory response is achieved through the replacement of the necrotized myocardium with granulation tissue represented by collagen scars (6). Although capable of maintaining heart function, collagen scars do not preclude HF afterwards massive MI. Since the extent of MI and the severity of remodelling depend on the inflammatory response of immune cells, several studies have shown the key role of innate immune cells in the post-MI response (7,8). In AMI patients, a series of mediators administers the inflammatory response through dynamically modulating the pro-inflammatory and anti-inflammatory reparative phases (9,10). During the last two decades ground-breaking research has identified small endosomal derived membrane microvesicles, known as exosomes (11,12). They are secreted by immune cells as the main interactors in the regulation of reparatory responses following prolonged ischemia (13). Many researchers in the field have fuelled their minds to develop new therapies aimed at modulating the immune response after AMI (14,15). In an interesting review written by Patil and colleagues (16), the central role that exosomes play in heart disease and therapy is pointed out. They underline an active post-MI role of exosomes derived from immune cells. In a recent issue of Circulation Research, Patil and colleagues also showed that awareness and improvement of the phagocytic function of immune cells is an important gain in understanding the pathophysiological mechanisms of repair (17). Specifically, they set up a pilot study aimed to verify whether and how “mesenchymal stem cell-derived exosomes (MSC-Exo) can act as opsonin for apoptotic cells and/or trigger ‘eat me’ phagocytic signaling in resident/recruited phagocytes after myocardial ischemic injury” (17).
Patil and colleagues used mouse MSCs and verified MSC-Exo-mediated opsonization of apoptotic cardiomyocytes. The authors then determined the mechanism by which MSC-Exo modulates the phagocytic activity of macrophages (Figure 1). The hypothesis proposed by the authors focuses on milk fat globule-EGF factor 8 (MFGE8), one of the main opsonins capable of binding apoptotic cells. The authors demonstrated an enrichment of MSC-Exo in MFGE8 protein and mRNA and verified the role of this factor in apoptotic cell clearance. They have shown both increased opsonization of dead cells and binding of MFGE8-presenting MSC-Exo to phagocytes which in turn induces activation of phagocytic signalling which increases their ability to remove dead cells.
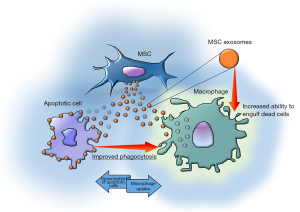
The authors claim the involvement of MSC-Exo in cardiac repair and their therapeutic potential in the improvement of heart function (18,19). They demonstrated in vitro the link between exosomes and apoptotic and necrotic H9c2, showing their ability to behave as opsonins towards apoptotic cells. They also showed the same ability towards cardiac fibroblasts. In both cases the bond is achieved through the interaction between MFGE8 and the phosphatidylserine (PS) exposed on the outer layer of the plasma membrane. It has also been verified that the phagocytic index significantly improved when H9c2 were exposed to the opsonization by MSC-Exo compared to the same non-exposed cells. In addition, an in vivo evaluation was performed to verify whether in these conditions the biological significance of the exosome-mediated opsonization had the same result on phagocytosis; therefore, by injecting apoptotic cardio-myoblasts into the mouse peritoneum, they discovered similar effects to the in vitro results. Nevertheless, in the complex study by Patil and colleagues, the most interesting finding may be considered the dual role of the MSC-Exo. Indeed, following the uptake in macrophages MSC-Exo are also able to activate intracellular signalling stimulating the efferocytosis, namely the biological process by which apoptotic cells are cleared by phagocytic cells (20).
Interestingly, and in accordance with the literature data (21,22), the authors highlighted an increase in the expression of integrin αVβ3 (MFGE8 receptor), an up regulation of its downstream RhoA and RAC1/2/3 and the inhibition of PTEN expression which regulates RAC expression levels. The biological significance of these molecular events ensures a transformation of the cytoskeleton, a rearrangement of actin, and facilitates the phagocytic activity of apoptotic cells (23).
Therefore, using a specific siRNA they produced MFGE8-knockdown (KD) MSC-Exo to evaluate the phagocytic activity of macrophages. Interestingly, they found that RAW 264.7 cells (monocyte/macrophage-like cells) used as a model, lost phagocytosis-related signalling. Intriguingly, the result described above was totally switched to a downregulation of both integrin αVβ3 and Rho-A and Rac 1/2/3 with an upregulation of PTEN. Furthermore, phagocytosis analysis revealed a reduced uptake of dead cells by macrophages pre-treated with MFGE8-KD MSC-Exo.
In light of the above, it can be said that the authors have clearly demonstrated that the biological effects of MSC-Exo on macrophage activity are largely dependent on the presence/absence of MFGE8.
The central goal formulated by the authors was further confirmed by the intramyocardial administration of MSC-Exo. Treatment with MSC-Exo immediately after left anterior descending (LAD) ligation and a second dose of MSC-Exo administered intravenously by injection into the tail vein 24 hours after surgery was performed. From these experiments, the in situ engulfment of dying heart cells, the infiltration of inflammatory cells, and the impact on remodelling and repair of tissues dependent on these phenomena were assessed.
The authors analyzed the results of this experimental set, 7 days after the surgical manoeuvres on heart sections. They assessed cTnT (cardiomyocyte marker) positivity within CD68+ macrophage cytoplasm and macrophage phagocytosis of apoptotic cells in myocardial tissue staining sections with the TUNEL assay kit. Observing an increase in the number of cytoplasmic cTnT-positive CD68+ cells and a significant increase in the number of TUNEL-positive CD68+ cells in the cytoplasm compared to mice that received PBS, post-MI.
Furthermore, in vivo intramyocardial administration of MSC-Exo revealed another interesting result regarding the resolution of inflammation, also in this case the administration of MSC-Exo significantly increased the clearance of dying cells, a result of great importance because it is associated with the downregulation of mRNA expression of myocardial pro-inflammatory cytokines such as iNOS, IL6 and CCL4, and upregulation of anti-inflammatory cytokine mRNA such as CCL24 and IL10. To corroborate these results, the authors also performed the evaluation of the neo-vascularization ascertained with staining for CD31+ cells and the evaluation of the functionality of the left ventricle by means of echocardiography. The results agree with all others supporting the evidence that MSC-Exo contributes to preserve heart function after myocardial ischemic injury, activating phagocytic macrophage signaling which promotes apoptotic cell clearance and reduces scar formation and fibrosis in the left ventricle. The latest analysis performed by the authors to verify whether myocardial repair and resolution of inflammation were mediated by MFGE8 was conducted by evaluating the effect on survival and remodelling of the left ventricle. This revealed that administration of MFGE8-deficient MSC-Exo failed to protect mice from MI complications.
As described by several authors the resident macrophages of the heart represent the tip of the balance in insult response, orchestrating the immune response and a series of events concerning cardiac repair, leading to a more or less compromised cardiac performance (24,25).
From the study by Patil and colleagues it is clear that the knowledge of exosomes is fundamental to improve pathological conditions that today lead in most cases to negative outcomes. This knowledge is key to developing new therapies that could have benefits for infarct patients.
Acknowledgments
Funding: The authors are supported by UNITO, RILO ex-60% and Fondo Beneficenza San Paolo (progetto B/2021/0159).
Footnote
Provenance and Peer Review: This article was commissioned by the editorial office, ExRNA. The article has undergone external peer review.
Conflicts of Interest: All authors have completed the ICMJE uniform disclosure form (available at https://exrna.amegroups.com/article/view/10.21037/exrna-22-1/coif). The authors have no conflicts of interest to declare.
Ethical Statement: The authors are accountable for all aspects of the work in ensuring that questions related to the accuracy or integrity of any part of the work are appropriately investigated and resolved.
Open Access Statement: This is an Open Access article distributed in accordance with the Creative Commons Attribution-NonCommercial-NoDerivs 4.0 International License (CC BY-NC-ND 4.0), which permits the non-commercial replication and distribution of the article with the strict proviso that no changes or edits are made and the original work is properly cited (including links to both the formal publication through the relevant DOI and the license). See: https://creativecommons.org/licenses/by-nc-nd/4.0/.
References
- Nowbar AN, Gitto M, Howard JP, et al. Mortality From Ischemic Heart Disease. Circ Cardiovasc Qual Outcomes 2019;12:e005375. [Crossref] [PubMed]
- Mechanic OJ, Gavin M, Grossman SA, et al. Acute Myocardial Infarction (Nursing). StatPearls. Treasure Island, FL, USA: StatPearls Publishing, 2021.
- Wang Y, Leifheit E, Normand ST, et al. Association Between Subsequent Hospitalizations and Recurrent Acute Myocardial Infarction Within 1 Year After Acute Myocardial Infarction. J Am Heart Assoc 2020;9:e014907. [Crossref] [PubMed]
- Berezin AE, Berezin AA. Adverse Cardiac Remodelling after Acute Myocardial Infarction: Old and New Biomarkers. Dis Markers 2020;2020:1215802. [Crossref] [PubMed]
- Zhang Y, Wen W, Liu H. The Role of Immune Cells in Cardiac Remodeling After Myocardial Infarction. J Cardiovasc Pharmacol 2020;76:407-13. [Crossref] [PubMed]
- Richardson WJ, Clarke SA, Quinn TA, et al. Physiological Implications of Myocardial Scar Structure. Compr Physiol 2015;5:1877-909. [Crossref] [PubMed]
- Frangogiannis NG. The inflammatory response in myocardial injury, repair, and remodelling. Nat Rev Cardiol 2014;11:255-65. [Crossref] [PubMed]
- Duncan SE, Gao S, Sarhene M, et al. Macrophage Activities in Myocardial Infarction and Heart Failure. Cardiol Res Pract 2020;2020:4375127. [Crossref] [PubMed]
- Pluijmert NJ, Atsma DE, Quax PHA. Post-ischemic Myocardial Inflammatory Response: A Complex and Dynamic Process Susceptible to Immunomodulatory Therapies. Front Cardiovasc Med 2021;8:647785. [Crossref] [PubMed]
- Ong SB, Hernández-Reséndiz S, Crespo-Avilan GE, et al. Inflammation following acute myocardial infarction: Multiple players, dynamic roles, and novel therapeutic opportunities. Pharmacol Ther 2018;186:73-87. [Crossref] [PubMed]
- Théry C, Witwer KW, Aikawa E, et al. Minimal information for studies of extracellular vesicles 2018 (MISEV2018): a position statement of the International Society for Extracellular Vesicles and update of the MISEV2014 guidelines. J Extracell Vesicles 2018;7:1535750. [Crossref] [PubMed]
- D'Ascenzo F, Femminò S, Ravera F, et al. Extracellular vesicles from patients with Acute Coronary Syndrome impact on ischemia-reperfusion injury. Pharmacol Res 2021;170:105715. [Crossref] [PubMed]
- Xiong YY, Gong ZT, Tang RJ, et al. The pivotal roles of exosomes derived from endogenous immune cells and exogenous stem cells in myocardial repair after acute myocardial infarction. Theranostics 2021;11:1046-58. [Crossref] [PubMed]
- Jung M, Dodsworth M, Thum T. Inflammatory cells and their non-coding RNAs as targets for treating myocardial infarction. Basic Res Cardiol 2018;114:4. [Crossref] [PubMed]
- Sid-Otmane C, Perrault LP, Ly HQ. Mesenchymal stem cell mediates cardiac repair through autocrine, paracrine and endocrine axes. J Transl Med 2020;18:336. [Crossref] [PubMed]
- Patil M, Henderson J, Luong H, et al. The Art of Intercellular Wireless Communications: Exosomes in Heart Disease and Therapy. Front Cell Dev Biol 2019;7:315. [Crossref] [PubMed]
- Patil M, Saheera S, Dubey PK, et al. Novel Mechanisms of Exosome-Mediated Phagocytosis of Dead Cells in Injured Heart. Circ Res 2021;129:1006-20. [Crossref] [PubMed]
- Zhao AG, Shah K, Cromer B, et al. Mesenchymal Stem Cell-Derived Extracellular Vesicles and Their Therapeutic Potential. Stem Cells Int 2020;2020:8825771. [Crossref] [PubMed]
- Wang X, Tang Y, Liu Z, et al. The Application Potential and Advance of Mesenchymal Stem Cell-Derived Exosomes in Myocardial Infarction. Stem Cells Int 2021;2021:5579904. [Crossref] [PubMed]
- Li Y, Li Q, Fan GC. Macrophage Efferocytosis in Cardiac Pathophysiology and Repair. Shock 2021;55:177-88. [Crossref] [PubMed]
- Torres-Gomez A, Cabañas C, Lafuente EM. Phagocytic Integrins: Activation and Signaling. Front Immunol 2020;11:738. [Crossref] [PubMed]
- Kloc M, Uosef A, Kubiak JZ, et al. Role of Macrophages and RhoA Pathway in Atherosclerosis. Int J Mol Sci 2020;22:216. [Crossref] [PubMed]
- Arienti S, Barth ND, Dorward DA, et al. Regulation of Apoptotic Cell Clearance During Resolution of Inflammation. Front Pharmacol 2019;10:891. [Crossref] [PubMed]
- Sanmarco LM, Eberhardt N, Ponce NE, et al. New Insights into the Immunobiology of Mononuclear Phagocytic Cells and Their Relevance to the Pathogenesis of Cardiovascular Diseases. Front Immunol 2018;8:1921. [Crossref] [PubMed]
- Lafuse WP, Wozniak DJ, Rajaram MVS. Role of Cardiac Macrophages on Cardiac Inflammation, Fibrosis and Tissue Repair. Cells 2020;10:51. [Crossref] [PubMed]
Cite this article as: Comità S, Penna C, Pagliaro P. The dual role of mesenchymal stem cell-derived exosomes modulates the phagocytic activity of macrophages and promotes cardiac recovery after heart attack. ExRNA 2022;4:10.